By their very nature, biological cells are extremely complex entities, with heterogeneity being seen in the smallest cell populations. An in-depth insight of what is happening in each of these cells at any given point can be obtained by analyzing each one individually. The anticipation of revealing novel information concealed within a single cell is inspiring researchers to develop innovative techniques for single-cell analysis.
Why is it crucial to investigate a single cell, then?
There are numerous benefits to studying cells at the single-cell level.
Heterogeneity: Cells within a tissue or population can be quite varied, showing different gene expression patterns and functional characteristics. This heterogeneity can be revealed by investigating cells at the single-cell level, allowing researchers to spot rare cell types, investigate cell growth and differentiation, and understand how cells respond to different stimuli.
Disease mechanisms and drug discovery: Many diseases are caused by cellular processes that are out of control or by the dysregulation of a certain cell type. Single-cell analysis helps to identify the specific cell types and molecular mechanisms involved in disease, as well as identify therapeutic targets and evaluate drug efficacy at a cellular level, enabling the development of more targeted therapies.
Systems Biology: Single-cell analysis can help identify the genes and regulatory networks that control the cellular and molecular processes governing tissue development and organogenesis. This can help to build more accurate models of biological systems and cellular processes, which could enhance our comprehension of complex biological processes and help identify the key drivers of diseases.
Precision medicine: Single-cell analysis has the potential to advance precision medicine, which strives to offer personalized treatments based on patient’s characteristics. Researchers can better understand disease heterogeneity and develop customized treatment plans for better patient outcomes by analyzing single cells from patients.
Techniques for studying single cells
There are several established techniques for isolating single cells from heterogeneous populations while preserving their biological integrity, and in recent years, innovative techniques with increased precision and accuracy are being developed.
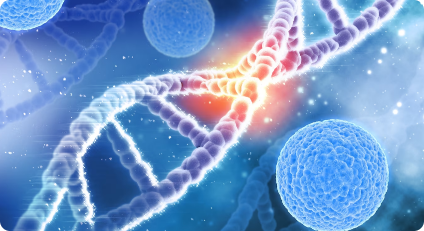
Immunohistochemistry (IHC): IHC is a technique used to detect specific proteins in tissues or cell samples. It involves labeling target proteins with specific antibodies that are conjugated to enzymes or fluorophores. The labeled antibodies bind to their target proteins, allowing for visualization and analysis of protein expression and localization at the single-cell level within tissue sections.
Flow Cytometry: In flow cytometry, cells are labeled with fluorescent markers specific to certain cellular characteristics, such as surface markers or intracellular proteins. The cells are then passed through a flow cytometer, which recognizes and measures fluorescent signals to reveal details about the physical and chemical properties of every single cell.
Imaging Techniques: Confocal microscopy, two-photon microscopy, and super-resolution microscopy are a few imaging techniques that can be used for single-cell research. These techniques can provide temporal and spatial information on protein localization and cellular processes.
Single-cell Sequencing: Single-cell sequencing involves isolating RNA, DNA, or both from individual cells and sequencing them. It provides crucial information about single-cell chromatin accessibility, genetic mutations, and gene expression, which helps shed light on cellular functions and mutations, and their implications.
Spatial Transcriptomics: Spatial transcriptomics involves the sequencing of RNA transcripts in situ, preserving the spatial information of the single cells within a tissue. It can be used to study cellular interactions and the spatial organization of tissues and helps researchers understand how genes are expressed in specific regions of complex biological systems.
Other techniques that researchers use include mass spectrometry, microfluidics, CRISPR-Cas9 screening, single-cell proteomics, single-cell epigenomics, single-cell metabolomics, and laser capture microdissection (LCM).
In general, these techniques can provide valuable insights into the properties and behavior of individual cells and can be used to study complex biological processes and disease mechanisms at a single-cell level.
Challenges in single-cell analysis
One of the greatest challenges for single-cell analysis is the inability to investigate the cells in their native environment to provide a complete picture of the cellular interactions. The viability, shape, and behavior of the cells are impacted by the isolation and processing that occurs in many of the single-cell analysis techniques currently being used. After being isolated, cells lose both the context of their spatial environment and the information of their state at the moment of their isolation.
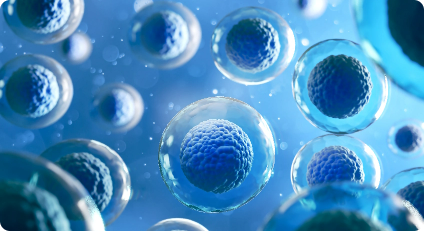
To be able to obtain precise and in-depth cellular insights while performing single-cell analysis, it is crucial to address these challenges.
Spatial Biology is an emerging field for single cell-analysis
Current single-cell analysis techniques have limitations in capturing the spatial organization and interactions of cells within tissues. Spatial biology addresses these challenges by integrating spatial information into single-cell analysis.
Spatial heterogeneity, or the presence of diverse cellular behaviors or characteristics in different parts of a tissue, is a common feature of biological processes and
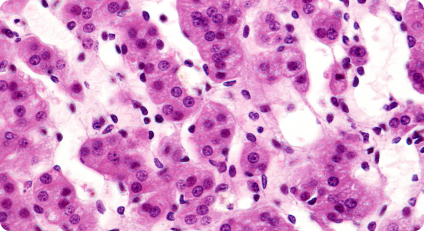
diseases. This spatial variation may go unnoticed in single-cell analyses. Spatial biology allows researchers to capture and analyze gene expression patterns within specific regions of interest, uncovering spatial heterogeneity and its functional implications.
Understanding the spatial distribution of cells and molecular alterations within diseased tissues is crucial for studying disease progression and developing targeted therapies. Spatial biology techniques can provide spatial maps of disease-related gene expression changes, spatially localized biomarkers, and the spatial distribution of pathological features, enhancing our understanding of disease mechanisms.
Spatial biology generates multidimensional data that combines spatial information with molecular profiles. Integrating these spatial datasets with single-cell transcriptomics or other omics data presents computational and analytical challenges. In order to properly utilize the sheer amount of information at our disposal, developing strategies for efficient data integration is an important objective in spatial biology.
Targeted Bioscience’s AccuLift Spatial Profiler System is designed to fulfil some of the most current and demanding needs of pathologists and researchers. It is an intuitive and powerful system that combines targeted spatial profiling with a cloud-based platform to provide a unique digital pathology ecosystem. With AccuLift, it is possible to profile targeted single cells of interest within the tissue microenvironment and use it for a variety of downstream applications such as oncology, neuroscience, single-cell profiling, proteomics and genomics. The innovations by Targeted Bioscience are helping to accelerate translational and clinical research to advance precision medicine.
To know more about Targeted Bioscience, click here.
References
- https://www.ncbi.nlm.nih.gov/pmc/articles/PMC3989949/#:~:text=The%20tissue%20microenvironment%20consists%20of%20a%20dynamic%20population%20of%20cellular,the%20homeostasis%20of%20an%20organ.
- https://www.ncbi.nlm.nih.gov/pmc/articles/PMC8818713/
- https://www.technologynetworks.com/cancer-research/articles/interrogating-the-complexities-of-the-tumor-microenvironment-367923
- https://www.biocompare.com/Bench-Tips/594803-How-to-Integrate-Spatial-Biology-into-Your-Research
- https://jbioleng.biomedcentral.com/articles/10.1186/s13036-017-0077-0#Sec11